Scientific testing of Zi-400 dates back to 1993, when the Australian Defence Force commissioned a study into the impact of 19 fully approved aviation cleaning products on the corrosion rates of aluminium alloy used in military aircraft.
The results speak for themselves. Zi-400 was found to be a powerful corrosion inhibitor, with the extraordinary ability to arrest existing corrosion after it has commenced, shutting down corrosion rates to almost zero.
These outstanding results were reproduced in a 2016 study conducted by Deakin University in Melbourne. You can read an in-depth summary of both reports below, or simply dive straight into the results.
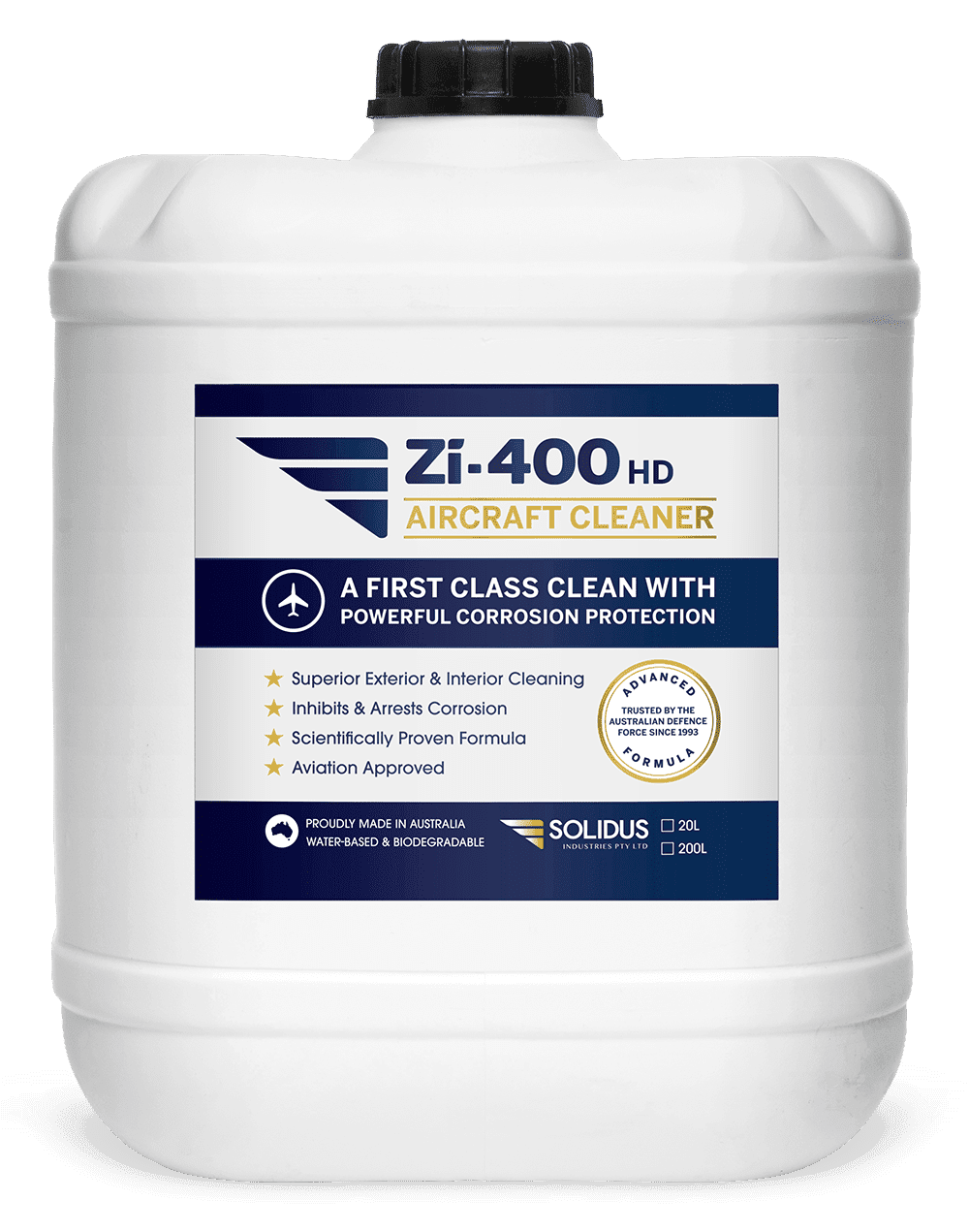
Introduction
Corrosion can be seen all around in our daily lives. The National Association of Corrosion Engineers (NACE) in the USA defines corrosion as “a naturally occurring phenomenon commonly defined as the deterioration of a material (usually a metal) that results from a chemical or electrochemical reaction with its environment”1.
A common example of corrosion is the rusting of steel (Figure 1).
Unfortunately, thermodynamics tells us that there is a natural tendency for most metals to return to their original state i.e. the ore from which they were processed (Figure 2). For example, steel reverts to iron ore (oxide or rust), and aluminium reverts back to aluminium oxide (Bauxite). These oxides are the result of corrosion. Therefore, corrosion is inevitable for most engineering alloys in many of the environments in which they are used. The challenge in the application and use of these alloys is to prevent them from corroding.
Why is corrosion a concern in air, marine and land based structures and assets?
Firstly, corrosion damage can reduce section thickness and therefore affect the mechanical integrity of structures through the initiation of fracture and fatigue cracking. This may lead to catastrophic failure and consequent loss of life.
Secondly, it is very expensive to find, remove and repair corrosion damage, and this adds to the through life costs associated with the maintenance of assets.
Thirdly, the time spent on dealing with corrosion represents a loss of capability of a ship, aircraft or land based structure, and the consequent loss of income derived from that asset.
The Corrosion Process
Figure 3. Schematic of the corrosion process
These local anodes and cathodes may be microconstituents in the alloy microstructure (Figure 4), or joints crevice and fastener holes in a structure (Figure 5).
In order for corrosion to occur, these local anodes and cathodes must be in contact with an aqueous environment or electrolyte e.g. a moisture layer containing salt contaminants. At the anode metal ions go into solution releasing electrons into the metal (the corrosion event). At the cathode, electrons are consumed by a cathodic reaction at the adjacent metal surface which may be the reduction of oxygen in the environment. Hydroxyl ions produced by this oxygen reduction reaction migrate to the anode through the electrolyte to maintain an electroneutral surface and an electrochemical circuit is established (Figure 3).
Break the circuit. Prevent corrosion.
Corrosion prevention is all about breaking this circuit or preventing it from becoming established. Either through preventing access of the environment to the surface, or introducing species into the environment which act at the local anodes and cathodes and prevent the reactions from occurring there.
This basic mechanism (Figure 3) may be applied to either pitting corrosion, crevice, intergranular, galvanic, or filiform corrosion, or stress corrosion cracking. This basic mechanism helps to understand how corrosion can be controlled or prevented (i.e. breaking the circuit), and is used to explain the effectiveness of various parts of a corrosion prevention strategy for aircraft structures.
The most common way to prevent corrosion from occurring is to apply a barrier coating (paint layer) over the metal surface to stop access of the environment. A paint coating not only acts as a barrier to moisture, it also provides a high resistance pathway to the movement of ions when the barrier has been permeated by water, thus restricting the flow of ions in the electrochemical circuit as depicted in Figure 3, and slowing down the corrosion process. In the event that a coating is damaged or deteriorates over time and fails mechanically, moisture can penetrate to the exposed metal substrate. Inhibitor pigments contained in the pre-treatment layers of paint coatings are available to provide corrosion protection. These inhibitors can act to prevent corrosion by shutting down potential electrochemical reactions at anodes and cathodes on surfaces and inside crevices, pits and cracks. Inhibitors may produce protective layers on the surface either by combination with the metal to form an oxide film for example, or by adsorption of molecules onto the surface.
Corrosion will not occur until the protective coating system fails. All paints on an aircraft structure will admit moisture over time, as they degrade due to weather exposure, UV, temperature excursions from high altitude flight, soil accumulation and stressing.
Evidence of degradation is discoloration, chalking and cracking. As they age and degrade they harden, and cracking develops particularly in areas of structure where flexing can occur e.g. within the joints, inside fastener holes and on mating surfaces. The network of cracks formed will allow all constituents of the atmospheric environment to penetrate through to the substrate metal. Inhibitor ions leached from the primer and conversion coating at the point of cracking will eventually be exhausted and corrosion will occur.
In addition to painting, another corrosion prevention and control strategy for corrosion protection and arrest is the use of the common maintenance action of periodic aircraft washing using detergent products. Washing of soil deposits from a surface removes potential local anodes and cathodes where the paint coating has failed. For example, a soil deposit can create a site for crevice corrosion. Washing not only washes away salt contaminants and dirt or soil, but the surface active nature of chemicals in detergents allows them to penetrate into cracks and joints where coatings have failed. The inclusion of an inhibitor into a detergent formulation when applied to the outside of the aircraft would find its way into cracks in coatings along joints and around fastener heads, and counteract the deleterious effect of any atmospheric contaminants such as chloride ions. These ions are very effective in initiating corrosion.
Laboratory Tests with Zi-400
Early research work in the 1980s at the Aeronautical Research Laboratories (now Defence Science & Technology Group (DST)) which provides science and technology support to the Australian Defence Force, showed that various surfactant compounds commonly used in detergent formulations were very good inhibitors of corrosion on aluminium alloys. This suggested to the DST researchers that many commercially available aircraft washing detergents may have corrosion inhibiting capabilities. In 1993 at the request of the RAAF, DST investigated 19 washing detergents for their ability to inhibit corrosion1. All of these products complied with various specifications including DEF (AUST) 5570A “Cleaning Compound, Aircraft Surface (Alkaline Waterbase)”, Boeing D6-17487 REVISION P (Exterior use), Boeing D6-7127 REVISION M (Interior use), AMS 1526B (Exterior use), AMS 1550B (Interior use). The tests called up in these specifications ensure the cleaning capability of the products, and ensure that the detergents do not corrode a range of metals at a greater than a specified rate. The specifications do not assess if the cleaner can actually inhibit corrosion, or slow the growth of existing corrosion.
The DST study concluded that Zi-400 was the most effective detergent for inhibiting corrosion in very severe corrosive environments, and most importantly it was capable of arresting or retarding existing corrosion. The study also showed that Zi-400 was very effective in reducing the rate of under film corrosion emanating from defects in an epoxy primer paint coating on an aluminium alloy, and reducing crevice corrosion on an aluminium alloy.
More recently, a comprehensive programme commissioned by Solidus Industries was carried out by Deakin University’s Australian Centre for Infrastructure Durability to undertake a more in depth assessment of how effective Zi-400 HD is as a corrosion inhibitor3. For comparative purposes tests were also conducted on a well-known brand of cleaner, widely used by the aviation industry and approved for use on aircraft (Product X). The main results from this programme are summarised in the remainder of this article. These results demonstrate how very effective Zi-400 HD is as a corrosion inhibitor.
Zi-400: The Results
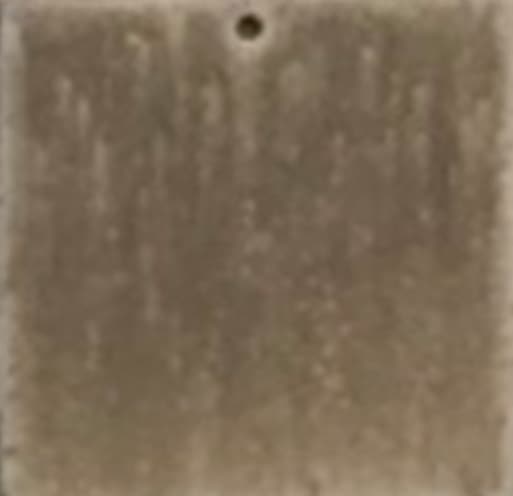
a. Control Solution 0.6% NaCl
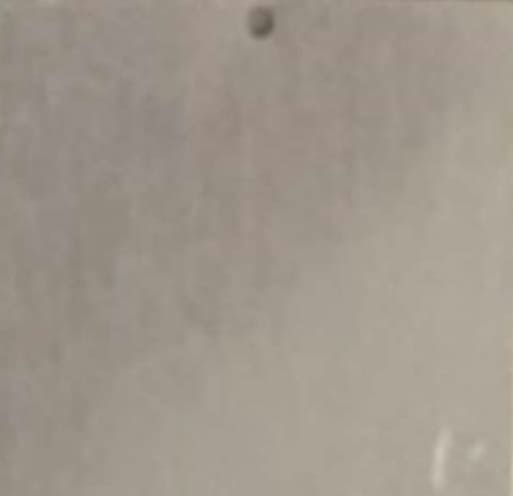
b. Control Solution with 3% Zi-400 HD
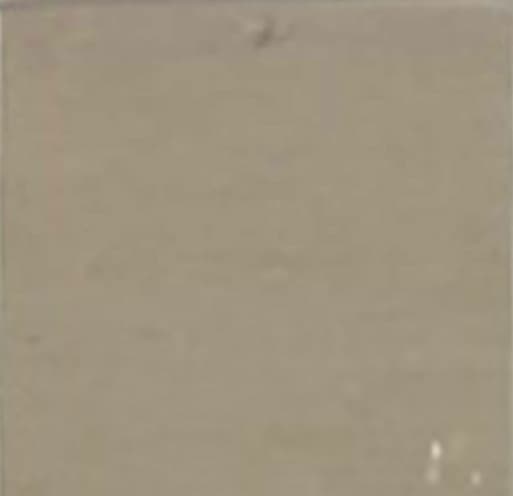
c. Control Solution with 17% Zi-400 HD
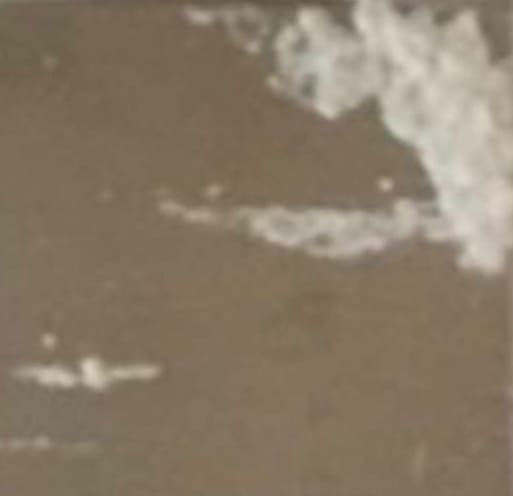
d. Control Solution with 3% Product X

e. Control Solution with 17% Product X
Figure 6. Specimens of aluminium alloy AA 2024-T351 after immersion for 30 days in chloride solution with and without Zi-400 HD and Product X.
Figure 6 shows the appearance of specimens of aluminium (Al) alloy AA2024-T351 after immersion for 30 days in a very corrosive Control solution of 0.6% sodium chloride, with and without the presence of Zi-400 HD and Product X. It is clear that after immersion the specimen exposed to the solution without Zi-400 HD was heavily corroded as indicated by the thick layer of aluminium corrosion product, which was associated with individual corrosion pits.
With both concentrations of Zi-400 HD present in solution, no corrosion product was present on the specimens after immersion. The dull appearance of the specimens was due to a very light surface etch. Similar results were observed with aluminium alloy AA7075-T651.
With Product X in the Control solution, severe localised corrosion occurred on the specimen with large amounts of corrosion product present. This corrosion attack was typical of exfoliation corrosion. Exfoliation corrosion is a type of localised corrosion that propagates along the grain boundaries of the elongated grain microstructure of aluminium alloy sheet, plate and extrusions parallel to the component surface. The corrosion products have a greater volume than the original alloy, so their presence creates stress at the grain boundaries, and this wedging action separates layers of metal causing severe delamination.
Exfoliation frequently occurs on aircraft structural aluminium alloy components. This result clearly illustrates the effectiveness of Zi-400 HD as a very effective corrosion inhibitor in an aggressive chloride environment even at low concentrations of 3%. It is important to note that the presence of the Zi-400 HD prevented pitting corrosion and exfoliation corrosion, which is a particular concern if it develops on aircraft structure, as it can lead to the initiation of fatigue cracking.
Using mass loss measurements, corrosion rates were obtained on all specimens after exposure for 30 days to the very corrosive environment. Data for AA2024-T351 are shown in Figure 7. These data confirm the visual observations and show that when Zi-400 HD was present in solution at 3% the corrosion rate was reduced by a factor of 3, and at 17% the corrosion rate was reduced by a factor of around 10. Similar reductions in rate were observed with AA7075-T651. Figure 7 clearly shows that with Product X present there was no inhibition effect and in the case of Product X at 3% the corrosion rate increased.
Rates of 0.001 mm/year (17% Zi-400 HD) indicate negligible corrosion, and they clearly demonstrate the dramatic ability of Zi-400 HD to prevent corrosion from occurring at a significant rate on aluminium alloys in a very corrosive environment.
Figure 7. Corrosion rate data for AA2024-T351 aluminium alloy in 0.6% NaCl solution (Control) and for additions of Zi-400 HD and Product X. The light blue bands represent the scatter obtained in the test data. NB. The Corrosion Rate axis is a log scale.
After cleaning the specimens, the maximum depth of corrosion across the specimen surface was measured using optical profilometry. Figure 8 shows data obtained for specimens of AA2024-T351. Corrosion pits up to a maximum of 47 µm were measured on the specimen immersed in the Control solution. It was difficult to measure the depth of the very light etching rather than pitting on the specimens exposed to the solution with Zi-400 HD at both concentrations, with depths of 3µm and less.
Figure 8. Maximum depths of corrosion measured on specimens of AA2024-T351 after immersion for 30 days in various solutions. NB: The y axis is a log scale.
Similar constant immersion corrosion tests were carried out for 30 days using SAE 4140 steel specimens. This steel is commonly used for high strength aircraft components. The visual appearances of these specimens are shown in Figure 9.
0.6% NaCl solution (Control)
17% Product X
17% Zi-400 HD
Figure 9. Specimens of SAE 4140 steel after immersion for 30 days in 0.6% NaCl solution (Control) with and without Zi-400 HD or Product X.
It is clear from these images that after 30 days in solution, the steel specimen in the Control solution was heavily corroded with a dense layer of black and red rust. With Zi-400 HD present very little corrosion was evident, and that corrosion was concentrated mainly around the hole used to suspend the specimen in solution. That is normal for a constant immersion corrosion test with steel. With Product X present, some corrosion also occurred around the hole but to a much greater extent and with further corrosion occurring as evidenced by the trail of rust away from the hole. It is clear that the presence of Zi-400 HD prevented pitting corrosion from occurring on the steel, and corrosion around the hole used for suspension of the specimen.
Figure 10. Corrosion rate data for SAE 4140 in 0.6% NaCl solution (Control) and for additions of Zi-400 HD and Product X. The light blue bands represent the scatter obtained in the test data.
The corrosion rate data obtained with the steel specimens are shown in Figure 10. It can be seen that at 3% the Zi-400 HD reduced the corrosion rate significantly, while at 17% the corrosion rate was reduced by a factor of around 6. With Product X present at 3%, the corrosion rate was reduced by a small but significant amount, and at a concentration of 17% the corrosion rate was reduced by a factor of 4. It is well known that high strength steels are very susceptible to corrosion in strong chloride environments. These results are a further demonstration of how effective Zi-400 HD is as a corrosion inhibitor.
Galvanic corrosion occurs where two dissimilar metals are in contact in a corrosive environment. The Galvanic Series is a thermodynamic table which shows the electrochemical potentials of metals and alloys in a sea water environment. These potentials provide an indication of how likely it is that corrosion could occur when dissimilar metals are in contact. Examples of some approximate potential values are given in Table 1.
Metal or Alloy (Anodes – Undergo corrosion) | Electrochemical Potential V sce |
Magnesium alloys | -1.60 – -1.67 |
Zinc | -1.00 – -1.07 |
Aluminium alloys | -0.76 – -0.99 |
Steel | -0.56 – -0.71 |
Copper | -0.31 – -0.40 |
Stainless Steels | -0.20 – -0.30 |
Silver | -0 .09 – -0.13 |
(Cathodes – Cause corrosion) |
Table 1. Approximate values of electrochemical potential for various metals in seawater.
The metals with very negative potentials will become anodes when in contact with metals with more positive potentials, which act as cathodes. An electrical current will flow between the anode and the cathode, and that current is related to corrosion rate through Faraday’s Law. For example, zinc undergoes galvanic corrosion when in contact with steel or copper. Galvanised steel is an example of galvanic corrosion where the zinc layer corrodes sacrificially to protect the underlying steel.
Dissimilar metal contact frequently occurs in structures where for example bolts or fasteners are used. A typical example would be steel rivets in aluminium aircraft structure. Sealants are typically used to prevent contact between the two dissimilar metals.
In the Deakin University study, a galvanic corrosion test was set up between aluminium alloy AA7075-T651 and copper (Cu) when both were connected and immersed in a 0.6% NaCl environment. As shown in Table 1, a potential difference of around 0.5V exists between these dissimilar metals. This represents a very strong driving force for corrosion of the aluminium alloy.
During immersion over 70 hours the current flowing between these two electrodes while immersed in the solution was monitored. The current is a measure of the corrosion rate on the aluminium alloy in this very aggressive environment.
Figure 11. Galvanic corrosion rates (AA7075-Cu couple) obtained after 70 hours immersion in a Control solution (0.6% NaCl) and with various additions of Zi-400 HD.
As shown in Figure 11, when the Zi-400 HD was present the galvanic corrosion rate on the aluminium alloy was reduced significantly. For example, with Zi-400 HD at 3 and 17%, a reduction in corrosion rate of around a factor of 4 was observed. These results show how effective an inhibitor of galvanic corrosion Zi-400 HD is in these very aggressive corrosive conditions. With 3% or 17% of Product X present a significant reduction in corrosion rate occurred also.
In a second series of tests, the galvanic corrosion between AA7075-T651 and Cu was allowed to proceed for 20 hours, and then Zi-400 HD and Product X was added to the control solution. The corrosion rates before the addition of the Zi-400 HD and after an addition are shown in Figure 12. It can be seen that after adding the Zi-400 HD the galvanic corrosion rate was reduced by between a factor of 3 or 4. This is a dramatic reduction in corrosion rate, and clearly demonstrates how effective Zi-400 products are in arresting or retarding an existing aggressive corrosion process. The addition of Product X at both concentrations had no effect on reducing the rate of galvanic corrosion.
Figure 12. Galvanic corrosion rates obtained between AA 7075-T651 aluminium alloys and copper when immersed in 0.6% NaCl solution before and after the additions of Zi-400 HD and Product X.
A galvanic corrosion test similar to that described above was conducted using aluminium alloy AA2024-T351 with Cu instead of AA7075-T651, and allowed to proceed for 20 hours before adding Zi-400 HD or Product X to the control solution. The results are shown in Figure 13. After adding the Zi-400 HD the galvanic corrosion rate was again reduced by between a factor of 3 or 4, further establishing the product’s exceptional ability to arrest an existing corrosion process. Significantly, the effect of adding Product X aircraft cleaner at both concentrations actually increased the galvanic corrosion rate. At a concentration of 17%, Product X increased the galvanic corrosion rate dramatically from 40 µA/cm2 to 74.5 µA/cm2, almost double the rate.
Figure 13. Galvanic corrosion rates obtained between AA 2024-T351 aluminium alloy and copper when immersed in 0.6% NaCl solution before and after the additions of Zi-400 HD and Product X.
Filiform corrosion (underfilm corrosion) is one of the most common forms of corrosion that can develop on aircraft structures, particularly on aluminium alloy components. It may occur at breaks or cracks in paint coatings and sealants, around fastener heads or along seams and joint lines. Figure 14 shows an example of filiform corrosion on an aircraft wing skin. It occurs where contaminants such as chloride salts are present in the environment and moisture in the form of condensation or high humidity. Corrosion proceeds along the interface between the coating and the underlying metal. The resulting corrosion products cause the coating to de-adhere (blister) producing a worm-like appearance on the surface (filiforms). This corrosion process may continue in the absence of external moisture, because chloride ions are able to migrate along the lines of filiform, and the hygroscopic nature of the chloride salt attracts moisture. If left unattended, the filiform corrosion may develop into intergranular and exfoliation corrosion particularly in fastener holes and this damage may initiate fatigue cracking with serious consequences.
Figure 14. Filiform corrosion propagating from cracked paint around a fastener head on the skin of an aircraft wing.
The 1993 DST study2 tested many different commercially available aircraft washing detergents for their ability to prevent filiform corrosion on an AA 7075-T651 aluminium aircraft structural alloy. Alloy specimens were coated with a clear epoxy polyamide film (50 µm dry film thickness) which did not contain any corrosion inhibitors. A 15 mm long scratch simulating a break in an aircraft coating was made through the coating to the substrate. One group of specimens were immersed in a 0.6% NaCl solution for 21 days, and another group was immersed in a NaCl containing 20% solution of Zi-400. Specimens were removed at various times and the progression of the under film corrosion which developed away from the scratch was monitored. The results are shown in Figure 15.
Figure 15. The penetration of underfilm (filiform) corrosion as a function of time in a solution of 0.6% NaCl with and without (Control) the addition of Zi-400.
Summary
It is clear from the Deakin University study that Zi-400 HD is a strong corrosion inhibitor for both aluminium alloys and steel, when present in a very corrosive chloride containing environment. Such environments are similar to those which can develop as thin layers of moisture on the external surfaces of aircraft and in marine structures. The study has also shown that Zi-400 HD is very effective in reducing the rate of galvanic corrosion which can occur between dissimilar metals in contact in moist corrosive environments. A most significant result of the study was that Zi-400 HD was able to dramatically reduce the rate of corrosion in an existing corrosion process to very low values. The early DST study found that Zi-400 was able to delay the initiation of filiform corrosion, and when it did initiate it propagated at a very slow rate.
As a result of the DST study, Zi-400 was shown to act as a cathodic inhibitor. That is it adsorbed strongly at cathodic sites (See Figure 3), reducing the rate of the cathodic reactions and thus reducing the rate of corrosion. This mechanism is thought to be the basis for the excellent corrosion inhibition performance of Zi-400.
The Deakin University study showed that washing detergent Product X which conformed to Boeing D6-17487 REVISION P and AMS 1526B aircraft specifications, when tested under identical conditions to those used for the Zi-400 HD tests, in some cases actually increased the rate of corrosion on both Al alloys and steel, when compared to the Control solution. It also had no effect on the corrosion rate when added to an existing corrosion process.
While regular washing of the outside of aircraft structures is mainly for the purpose of cleaning away deposited soil and airborne contaminants such as chloride, these studies have shown that washing with a detergent such as Zi-400 HD also has the effect of applying a powerful corrosion inhibitor to the outside surfaces. The surface-active properties of Zi-400 HD will allow it to penetrate into cracks in paint coatings, into fastener holes and into joints and crevices, where it can provide significant protection against corrosion. The data shown above indicate that the effectiveness of Zi-400 HD increases with increasing concentration. With regular washing and rinsing, it would be expected that concentrations of Zi-400 HD would build up within fastener holes, joints and crevices, thereby providing an ongoing level of protection. The added benefit of washing with Zi-400 HD is that if corrosion had already started in those locations, this product would act to significantly reduce the rate at which the corrosion progresses. This was strongly indicated by the galvanic corrosion tests where Zi-400 HD was added to the corrosion environment after corrosion had initiated.
All the tests performed clearly show Zi-400 HD has the unique ability to control corrosion. This reduction in corrosion would be evident in the field wherever Zi-400 HD is regularly used as part of a corrosion prevention and control programme across a wide range of industries from aviation, marine, power generation, military, oil & gas, mining and industrial.
Conclusions
- Zi-400 HD has been shown in the Deakin University study to be an excellent inhibitor of corrosion of aluminium alloys and steel in a very corrosive chloride containing environment.
- Its effectiveness as an inhibitor has been demonstrated by its prevention of pitting corrosion under constant and alternate immersion conditions, filiform corrosion, crevice corrosion and galvanic corrosion.
- Zi-400 has the ability to retard and arrest the growth of a corrosion process under very severe conditions. This is of particular value where the corrosion process is driven by the interaction of dissimilar metals providing a very strong driving force for corrosion.
- Zi-400 is able to provide excellent corrosion inhibition because it is thought to adsorb strongly at local cathodes on the surface thus reducing significantly the rates of cathodic reactions, and therefore retarding the overall electrochemical corrosion process.
References
- G. A. Jacobson, Corrosion – A natural but controllable process, https://www.nace.org/resources/general-resources/corrosion-basics
- B. R. W. Hinton, M. Cosgrave, P. Rohan and D. Luscombe, Corrosion Inhibition with Aircraft Washing Detergents, Commercial in Confidence, ARL Report No. CCR 7/93, May, 1993.
- P. Huang, J. Yan, T. Khoo and B. Hinton, Corrosion Inhibition with Aircraft Washing Detergents, Deakin University, Australian Centre for Infrastructure Durability Technical Report No. T-C0098-1, 5 August 2016.
Contact Solidus Industries to get a quote on Zi-400 for your industry to protect your valuable assets against corrosion.
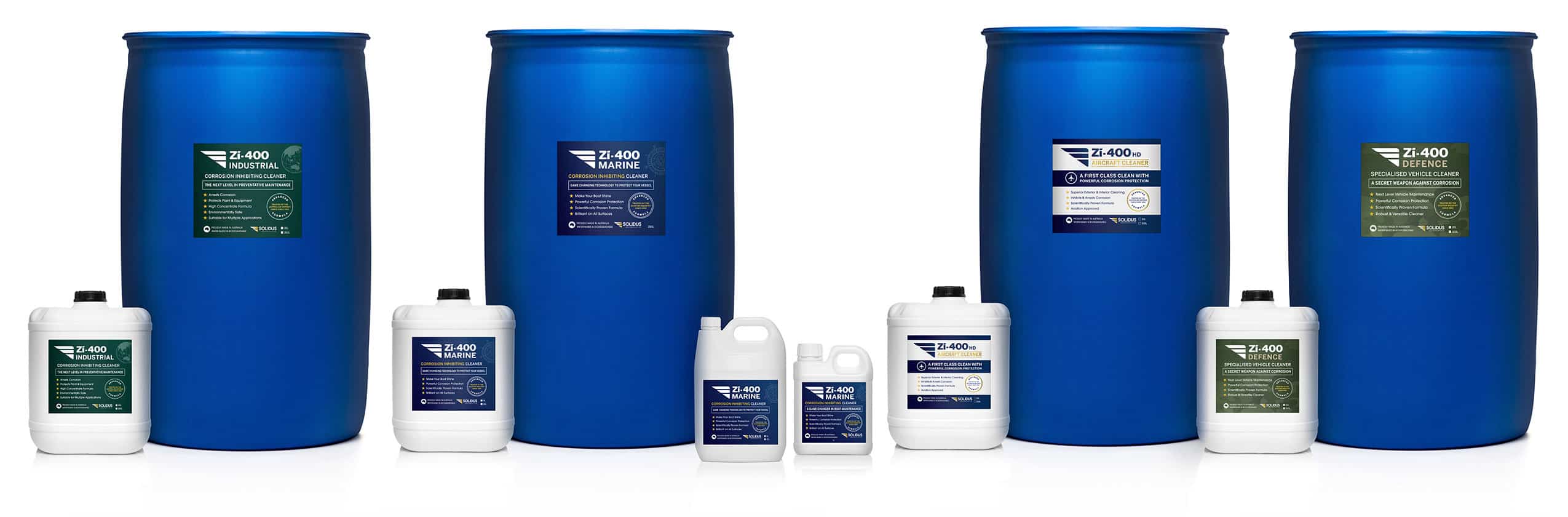
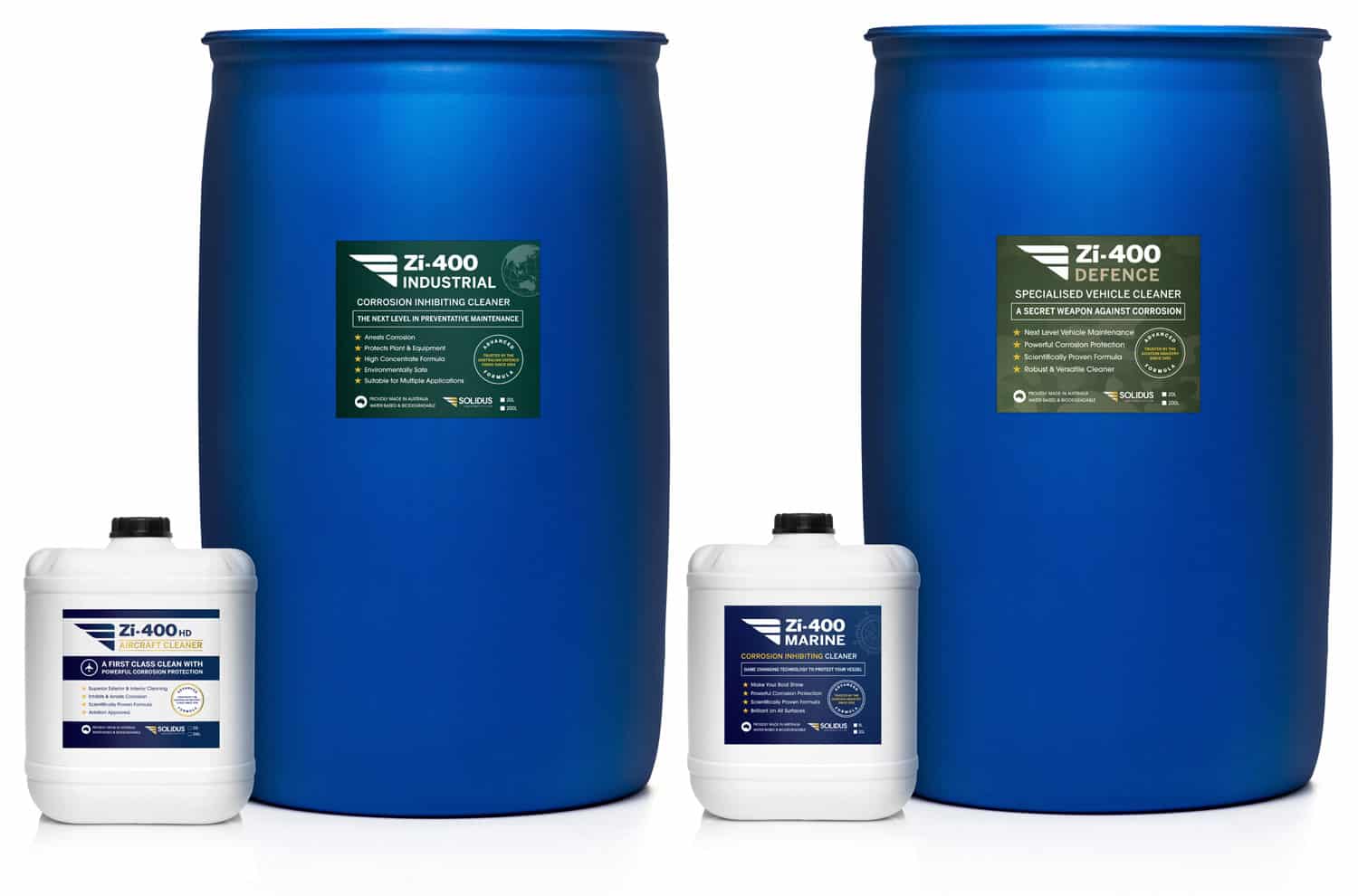